Dibble Glacier, Icebergs and Sea Ice.
by Ben Holt and Ron Kwok
The ability to derive quantitative estimates of fine-scale ice motion and deformation from pairs of Seasat SAR images provided the primary scientific basis for implementing ASF. Being able to identify the detailed opening and closing of leads and how the ice cover moved and deformed over short time intervals provided basic knowledge of air-sea-ice heat and momentum fluxes. The value of such detailed measurements was a primary motivation of the Arctic Ice Dynamics Joint Experiment (AIDJEX) that took place in the Beaufort Sea in the mid-1970s, where an extensive suite of on-ice plus airborne and satellite (in that case Landsat and NOAA) remote sensing observations were employed to determine external forcing that impacted the mechanical deformation and heat balance of the ice cover, which in turn could be used to verify and improve various types of sea-ice models.
With the Arctic sea ice imagery obtained from Seasat in 1978, a means of making such detailed ice motion observations under all conditions was possible, as first described using Seasat SAR optically processed imagery (Hall and Rothrock, J. Geophysical Research,1981). When Frank Carsey arrived at JPL in 1981, where the Seasat project and data were located, he set out to examine the Seasat instrument suite including the SAR imagery. Carsey hired one of us (Holt) to help him out with his research; Holt having freshly completed the Seasat SAR Ocean and Sea Ice Atlas (Fu and Holt, JPL Publication 81-120, 1982). They scrounged together a few pairs of digitally processed SAR images that contained the same ice fields taken 3 days apart. The Seasat SAR digital processor was the first ever developed and at that time a single 100-km by 100-km SAR image frame took no less than 8 hours to process, if it was done correctly the first time. With the help of some clever folks in the JPL Image Processing Lab, a method was established of interactively tracking specific floes from image to image and quantifying the motion. Carsey invited Drew Rothrock and Alan Thorndike, both from the Applied Physics Laboratory at the University of Washington, down to JPL and everyone sat down for a week or so to tediously complete the detailed floe tracking of these sets of image pairs. The geometric fidelity of the Seasat SAR was quite good and the floes could be identified nicely from image to image with its fine resolution. Thus, a clear, unambiguous, detailed, repeatable and useful geophysical quantity was derived. This was in contrast to the detailed and rich array of ocean features detectable by SAR, including waves, internal waves, eddies, currents, and fronts, but whose value were fraught by pesky and significant non-linearities arising from ocean motion during the imaging process and the even now tricky part of how to turn these fascinating signatures of the ocean surface into meaningful quantities.
A small working group was setup by NASA to consider establishing a SAR receiving station at UAF for ESA’s ERS-1 mission. This effort led to the initial science requirements document, Science Program for an Imaging Receiving Station in Alaska, published at JPL in December 1983. Some of the Seasat image pairs and motion vectors were prominently displayed and discussed in the report along with a broad spectrum of other important science topics, describing the wonderful things that could be done if we only had a receiving station to get this data regularly and that UAF was the best place to put it, both in terms of satellite accessibility and scientific interest. Published papers followed that discussed the derived vectors and their value as well as the geometric basis and accuracy of the measurements (Curlander, et al., IEEE J. Oceanic Eng., 1985; Carsey and Holt, J. Geophysical Res., 1987). A more complete Science Plan for the Alaska SAR Facility Program was published in 1989, following two highly entertaining and useful science meetings held at Chena Hot Springs, Alaska, in 1987 and 1988.
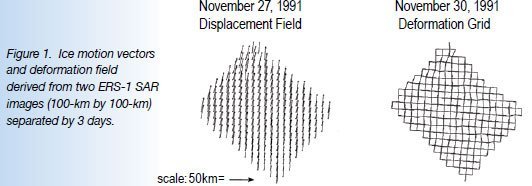
A brief mention should be made of a parallel effort to derive ice type from the SAR backscatter. Ice type is a proxy observation for the still difficult-to-measure ice thickness, so reliable methods to identify ice type would provide a means to assess the varying composition of the major ice types (and then thickness). As with most surfaces, using a sensor response to clearly identify a surface type or feature is often ambiguous. If it’s not the instrument properties itself, then it’s the variation in the surface that muddles the signatures. Another workshop, entitled the Radar Age/Type Algorithm Group (RAGTAG), was held in Seattle in July 1988 to explore ways to identify ice types. One thing that was clear, was that this could be done better using C-band, with the upcoming ERS-1 SAR and, on the more distant horizon, RADARSAT-1, than with Seasat L-band SAR, and that the major thicker ice categories could be pretty well identified with backscatter. The muddling part was that the thinner ice types sometimes had signatures similar to those of the thicker ice types and confusion ensued. This effort to derive ice type was continued within the GPS development discussed below. But then the notion of Lagrangian tracking with RADARSAT-1 basically leapfrogged the issue of ambiguous ice signatures to jump straight into ice age, a more desirable and valuable quantify from which ice growth and volume increase could be determined.
Implementation and products
At the time of the IMAG meetings, John Curlander (who headed the JPL SAR data processing group) and Carsey proposed to put together an operational system for producing sea-ice motion at ASF. The second author (Kwok) happened on the scene at around the same time — he was in Curlander’s group working on the Venus radar and was asked whether the work would be of interest. Without experience in sea-ice remote sensing, Kwok got involved and was given the responsibility to refine the algorithms and to work with VEXCEL (Franz Leberl was Chief Executive Officer) in constructing a system to be delivered to ASF sometime before the launch of ERS-1. The system incorporated the best of the various methods from the IMAG group, plus some new bells and whistles, and the Geophysical Processing System (GPS) development was initiated. The implementation effort was monitored by a group called the “Plumbers” (coined by R. Thomas) who were tasked with making sure that the image matching schemes and system had the correct “plumbing” or design. Numerous meetings were held at the new facilities of the then young VEXCEL in Boulder, Colorado.
The GPS system was a rather modest system by today’s standards (Kwok, et al., IEEE. J. Oceanic Engineering, 1990). It produced motion estimates from ~10 ERS image pairs daily and demonstrated the concept of producing geophysical information directly from SAR imagery. Processing speed was limited by the array processors of ~100 Mflops. There are only one-to-two – hundred vectors from each image pair (Fig. 1). Also, the production of the vectors required some attention because we were not familiar with the extent and range of deformation that one could expect within a single SAR image over 3 days. The GPS processed most of the ERS-1 SAR data of sea ice downlinked at ASF. The system was retired 3 years after it was installed.
The promise of routine coverage of the entire Arctic Ocean ice cover came with the RADARSAT-1 mission. The RGPS (RADARSAT Geophysical Processor System) was implemented to take advantage of its wide-swath mapping capability — the ScanSAR mode provides complete maps of the Arctic Ocean every 3 days, termed the Arctic Snapshot. This frequent and reliable coverage allowed us to follow drifting ice parcels for an entire winter and to record their evolving properties (i.e., deformation and ice age) in addition to ice motion on a uniform grid. Thus, the Lagrangian tracking approach was introduced. This idea was developed during a meeting of the second author with Drew Rothrock and Harry Stern at the University of Washington (Kwok, et al., IEEE Trans. Geosci. Remote Sens., 1995).
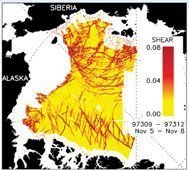
The RGPS system was funded, built, and began operations at ASF in 1999. At this writing, both ASF and JPL are involved in a joint project to process the ongoing Arctic Snapshot data stream acquired by RADARSAT-1, which started in the winter of 1996/97.
More than a thousand motion vectors can be obtained from a single RADARSAT-1 image pair. The quality of the motion trajectories is comparable to that from drifting buoys – the only remaining advantage of buoy observations being that of temporal sampling. RGPS observations are nearly equivalent to deploying thousands of drifting buoys in the Arctic Ocean. The basin scale maps of deformation are quite spectacular, providing detailed information about the response of the ice cover to atmospheric and oceanic forcing. Large-scale gradients in these forcings are clearly concentrated at the small scale in fracture zones where most of the dynamic and thermodynamic interactions between the atmosphere and ocean take place (Figure 2). This data set has given new impetus to process/climate studies and model improvement. The hope is that RADARSAT-2 will allow us to continue to build up a new decadal
record of observations at this scale in the years to come.