SAR: The Power Tool of Remote Sensing
Synthetic aperture radar (SAR), used to create the majority of the imagery available in the ASF archive, is one of the power tools of remote sensing. Synthetic aperture radar (SAR) bounces a microwave radar signal off the Earth’s surface to detect physical properties.
YouTube video courtesy Adrian Schubert
Through Snow and Rain and Dark of Night
Unlike optical technology, synthetic aperture radar (SAR) can “see” through darkness, clouds, and rain, detecting changes in habitat, levels of water and moisture, effects of natural or human disturbance, and changes in the Earth’s surface after events such as earthquakes or sinkhole openings.
Applications
Synthetic aperture radar (SAR) has been used in a wide range of applications, from studying Antarctic icebergs, to tracking the paths of oil spills into sensitive marshes, to mapping the wetlands of Alaska.
SAR Applications
Discover examples of how researchers around the world are using SAR
Basic SAR Concepts and Terminology
Also see: Synthetic Aperture Radar (SAR) and InSAR Guides.
How Does it Work?
A synthetic aperture radar (SAR) is an active sensor that first transmits microwave signals and then receives back the signals that are returned, or backscattered, from the Earth’s surface.
Flight and Directional Terminology
The instrument measures distances between the sensor and the point on the Earth’s surface where the signal is backscattered. This distance is slant range (see illustration), which can be projected on the ground representing the ground range. The flight direction is also referred to as along-track or azimuth direction, and the direction perpendicular to the flight path is the across-track or range direction. The angle between the direction the antenna is pointing and the nadir is the look angle. The angle between the radar beam center and the normal to the local topography is the incidence angle. Both angles are sometimes used synonymously, which is only valid if the InSAR geometry is simplified neglecting the Earth’s curvature and the local topography. Because the look angle of the sensor significantly affects the behavior of backscatter, it is one of the main parameters determining the viewing geometry and the incidence angle of the backscattered signal. Depending on the characteristics of the illuminated terrain, areas of layover and shadow may occur in the imagery.
Wavelength and Effects
The wavelength of the sensor determines the penetration depth of the transmitted signal into the vegetation layer of the terrain surface. The longer the wavelength, the deeper the penetration can be, particularly in forests.
- The energy of an X-band sensor is mainly returned at the top layer of the canopies
- Most of the L-band signal penetrates through the upper vegetation layer and is returned at the ground surface.
- The backscatter behavior of C-band is less predictable. Due to volume scattering effects, the layer of backscattering is less determined and does not correspond directly to a terrain surface — neither the vegetation surface nor the ground surface.
A typical side-looking radar pointing perpendicular to the flight direction. Credit: NASA.
Resolution and Speckle
The spatial resolution of the radar sensor defines the minimum separation between the measurements the sensor is able to discriminate and determines the amount of speckle introduced into the system. Speckle is a scattering phenomenon that arises because the spatial resolution of the sensor is not sufficient to resolve individual scatterers. Speckle can be reproduced if the acquisition conditions are identical, while noise is random in nature. Speckle is removed by multi-looking. The higher the spatial resolution of the sensor, the more objects on the ground can be discriminated. The term spatial resolution is often confused with the pixel size, which is the spacing of the pixels in the azimuth and ground range direction after processing the data.
SAR Frequently Asked Questions
See Sentinel-1 FAQ.
Unlike the aperture in a camera, which opens to let in light, radar aperture is another term for the antenna on the spacecraft or aircraft. The radar antenna first transmits electromagnetic energy toward Earth and then receives the returning energy after it reflects off of objects on the planet. In the NASA image below, the radar antenna is the rectangle at the Earth end of the 1978 Seasat satellite. The data collected by the radar antenna are then transmitted to another kind of antenna on Earth — such as the antennas of the ASF Satellite Tracking Ground Station — so they can be stored and processed.
In general, the larger the antenna, the more unique information scientists can obtain about an object — and the more information, the better the image resolution. However, antennas in space are not large. So scientists use the spacecraft’s motion, along with advanced signal-processing techniques, to simulate a larger antenna.
Synthetic aperture radar (SAR) interferometry (InSAR) detects motion or elevation by comparing radar signals from two or more images of the same scene. The images are taken at different times from the same vantage point in space. SAR interferometry is often used to detect surface changes (for use in seismology, for example) or to generate digital elevation maps. The InSAR image below shows deformation on Okmok, a volcano in the Aleutian Islands. Image courtesy of Zhong Lu, © ESA 2008.
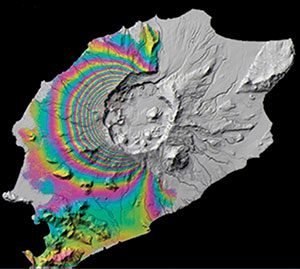
Because the radar wavelength is longer than particles in a cloud, such as droplets, the signal traveling through a cloud is mostly unaffected by any refraction at the boundaries of the different media.
In microwave remote sensing, scientists measure the time and magnitude of the signal backscattered from the ground to the radar antenna. The magnitude of the signal defines the brightness of a given pixel in the image. The resulting image has a grayscale. Scientists sometimes colorize SAR images to highlight certain data or features.
The interpretation of synthetic aperture radar (SAR) images is not straightforward. The reasons include the non-intuitive, side-looking geometry. Here are some general rules of thumb:
- Regions of calm water and other smooth surfaces appear black (the incident radar reflects away from the spacecraft).
- Rough surfaces appear brighter, as they reflect the radar in all directions, and more of the energy is scattered back to the antenna. Rough surface backscatter even more brightly when it is wet.
- Any slopes lead to geometric distortions. Steeper angles lead to more extreme layover, in which the signals from the tops of mountains or other tall objects “lay over” on top of other signals, effectively creating foreshortening. Mountaintops always appear to tip towards the sensor.
- Layover is highlighted by bright pixel values. The various combinations of the polarization for the transmitted and received signals have a large impact on the backscattering of the signal. The right choice of polarization can help emphasize particular topographic features.
- In urban areas, it is at times challenging to determine the orbit direction. All buildings that are perfectly perpendicularly aligned to the flight direction show very bright returns.
- Surface variations near the size of the radar’s wavelength cause strong backscattering. If the wavelength is a few centimeters long, dirt clods and leaves might backscatter brightly.
- A longer wavelength would be more likely to scatter off boulders than dirt clods, or tree trunks rather than leaves.
- Wind-roughened water can backscatter brightly when the resulting waves are close in size to the incident radar’s wavelength.
- Hills and other large-scale surface variations tend to appear bright on one side and dim on the other. (The side that appears bright was facing the SAR.)
- Due to the reflectivity and angular structure of buildings, bridges, and other human-made objects, these targets tend to behave as corner reflectors and show up as bright spots in a SAR image. A particularly strong response — for example, from a corner reflector or ASF’s receiving antenna — can look like a bright cross in a processed SAR image.
In ASF’s full-resolution synthetic aperture radar (SAR) images, objects can be distinguished as small as about 30 meters wide. Some of the smaller items scientists have spotted have been ships and their wakes. When the synthetic aperture radar (SAR) happens to be aligned at a certain angle, long thin objects such as roads or even the Alaskan oil pipeline can also be seen. Objects can be much smaller than the resolution and still be observable such as bright point objects. They only need to be perfectly aligned with the look direction of the synthetic aperture radar (SAR) sensor.
As the spacecraft moves along in its orbit, the radar antenna transmits pulses very rapidly in order to obtain many backscattered radar responses from a particular object. The synthetic aperture radar (SAR) processor could use all of these responses to obtain the object’s radar cross-section (how brightly the object backscattered the incoming radar), but the result often contains quite a bit of speckle. Generally considered to be noise, speckle can be caused by an object that is a very strong reflector at a particular alignment between itself and the spacecraft, or by the combined effect of various responses all within one grid cell. To reduce speckle, the data are sometimes processed in sections that are later combined — called looks. The more looks used to process an image, the less speckle. However, resolution is reduced, and information is lost in this process. Several research groups are developing/improving algorithms to reduce speckle while saving as much accurate information as possible.
Noise is defined as random or regular interfering effects that degrade the data’s information-bearing quality. Speckle is a scattering phenomenon that arises because the resolution of the sensor is not sufficient to resolve individual scatterers. Physically speaking, speckle is not noise, as the same imaging configuration leads to the identical speckle pattern. Speckle is removed by multi-looking. See “What is a ‘look'” above.
After the radar sends its microwave signal toward a target, the target reflects part of the signal back to the radar antenna. That reflection is called backscatter. Various properties of the target affect how much it backscatters the signal.
IfSAR is another term for InSAR. InSAR is the more common term, particularly for satellite-borne sensors. IfSAR has been used more by the military and/or for airborne sensors.
Consult ASF’s Get Started page.
Layover is a type of distortion in a synthetic aperture radar (SAR) image. The radar is pointed to the side (side-looking) for imaging. Radar signals that return to the spacecraft from a mountaintop arrive earlier or at the same time as the signal from the foot of the mountain, seeming to indicate that the mountaintop and the foot of the mountain are in nearly the same place, or the mountaintop may also appear “before” the foot. In a synthetic aperture radar (SAR) image with layover, the mountains look as if they have “fallen over” towards the sensor.
Where features are shifted from their actual location, the resulting geolocations are incorrect. This effect can be removed by the technique of terrain correction (also see “What is terrain correction?” below).
As with shadows from sunlight, shadows in synthetic aperture radar (SAR) images appear behind vertical objects. Mountains may appear to have black shadows behind them, depending on the steepness of the slope. The shadows appear black because no radar signals return from there.
Radiometric correction involves removing the misleading influence of topography on backscatter values. For example, the correction eliminates bright backscatter from a steep slope, leaving only the backscatter that reveals surface characteristics such as vegetation and soil moisture.
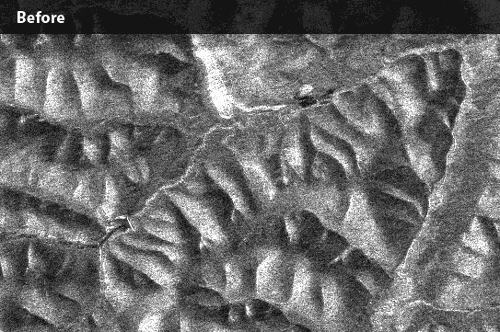
© JAXA/METI 2008.
Terrain correction is the process of correcting geometric distortions that lead to geolocation errors. The distortions are induced by side-looking (rather than straight-down looking or nadir) imaging, and compounded by rugged terrain. Terrain correction moves image pixels into the proper spatial relationship with each other. Mountains that look as if they have “fallen over” towards the sensor are corrected in their shape and geolocation.
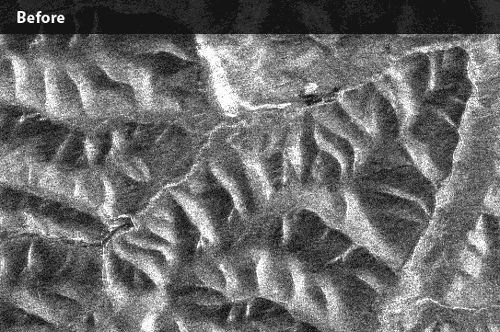
Material © JAXA/METI 2008.
The RTC products ASF generated for the ALOS PALSAR mission include a copy of the DEM used to process the RTC. Note that this DEM is not generated from current SAR data, but is a geoid-corrected version of the best publicly-available DEM for the area covered by the RTC product. Sources vary depending on the area, but generally use either the SRTM (Shuttle Radar Topography Mission) or 3DEP (3D Elevation Program, including seamless products formerly called NED) DEMs.
For more information on the DEMs included in the RTC product, visit the ALOS PALSAR RTC page.
Most digital elevation models (DEM) are geoid-based and require a correction before they can be used for terrain correction. The DEM included in an ASF radiometrically terrain corrected (RTC) product file was converted from source DEM orthometric height to ellipsoid height using the ASF MapReady geoid_adjust tool. This tool applies a geoid correction so that the resulting DEM relates to the ellipsoid.
An online tool is available that computes the height of the geoid above the WGS84 ellipsoid, and will show the amount of correction that was applied to the source DEM used in creating an RTC product.
Orthorectification corrects geometric distortions in imagery, just as terrain correction does (see “What is terrain correction?” above). The term ‘orthorectification’ is used more often in association with aerial and optical imagery. Terrain correction generally refers to synthetic aperture radar (SAR) imagery.
A georeferenced image has the location of the four corners of the image and the information needed to put the data into a projection. Geocoded data is already projected. Each point in the image is associated with a geographic coordinate.
C-band (~5.3 GHz)
Applies to ERS-1, ERS-2, RADARSAT-1, Sentinel-1
– Variety of applications, but particularly sea ice, ocean winds, glaciers.
L-band (~1.2 GHz)
Applies to PALSAR, UAVSAR, AIRSAR, JERS-1, Seasat
– Provides vegetation penetration
– Applications included sea ice, tropical forest mapping, soil moisture
– Subject to ionospheric effects
P-band (~0.4 GHz)
Applies to some products of UAVSAR
– Greatest penetration depth through vegetation and into soil
– Ideal for soil moisture and biomass
– Difficult to operate from space due to ionospheric effects
SAR Reference Guides
Fundamentals of Remote Sensing
Natural Resources Canada – Online comprehensive guide: concepts, image interpretation, applications. Clear writing, helpful illustrations.
Earth Observation College
Highly Recommended. Online In-depth comprehensive lessons and tutorials from concepts to applications.
ASF SAR User’s Guide
In 1993, when ASF was still named the Alaska SAR Facility, ASF produced a definitive synthetic aperture radar (SAR) User’s Guide that scientists continue to use today. Dense text, equations.
NOAA SAR Marine User’s Manual
The 2004 NOAA synthetic aperture radar (SAR) Marine User’s Manual, which starts with principles of synthetic aperture radar, is 464 pages long, with over 240 images and figures.
Radar and SAR Glossary
Comprehensive European Space Agency (ESA) list of synthetic aperture radar (SAR) terms and concepts, with figures.
The ASAR User Guide
ESA’s guide to the Advanced Synthetic Aperture Radar (ASAR) instrument on the ENVISAT mission includes a great deal of widely relevant content, including a Scientific Background section.
InSAR Reference Guides
GMTSAR: An InSAR Processing System Based on Generic Mapping Tools
2011 guide for an open-source InSAR processing system designed for users familiar with Generic Mapping Tools; the GMTSAR system is available here.
Delft Object-oriented Radar Interferometric Software User’s manual and technical documentation
2008 technical documentation and information for Delft open-source software, one of many options for processing InSAR.